Ten years of gravitational-wave detections
We spoke with Michele Vallisneri about his recent move to ETH Zurich, his research plans and the profound impact of the first gravitational-wave detection in 2015.
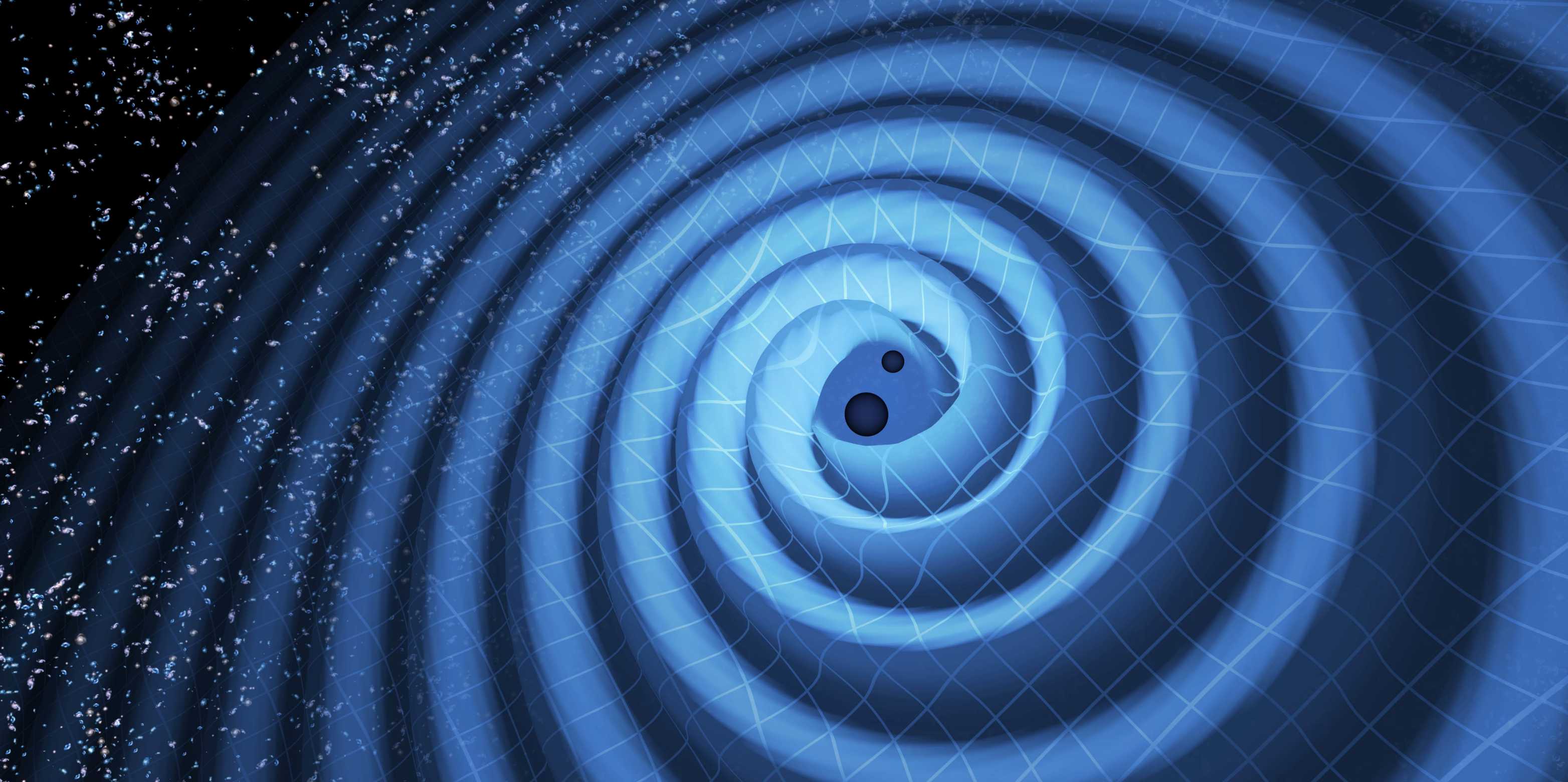
Michele Vallisneri is Professor of Gravitational Physics in the Department of Physics at ETH Zurich, where he's also involved in the activities of ETH Space.
How have you been settling in at ETH Zurich?
I've been here since January, and I currently have a PhD student and a postdoctoral researcher. Another student and two more postdocs will arrive in the next two or three months, so we'll soon be even more of a research group. We'll also be closely linked to ETH Space through my role as director of the Swiss Data Centre for the external page Laser Interferometer Space Antenna (LISA) space mission: this means there will be programmers who won't be here physically but will be part of the group's activities.
Since I arrived, I've been impressed by the professionalism of the colleagues I met in the department and elsewhere at ETH. Initiatives like teaching support and faculty leadership training seem very positive to me, and the generous resources that are available for doing research give professors the chance to build a group, hire students and staff before having to apply for research grants. And there's the feeling of being part of an important mission here, considering the pivotal role of ETH Zurich in the Swiss society.
I also found Zurich to be a young, multilingual city – and I like going around by tram! My wife Elisa Piccio, who's an archivist, my son Luca and my daughter Clara will join me this summer. They're excited to be back in the 'old world' among mountains, lakes and history, after a lifetime in California. Luca can't wait to explore Zurich's mountain bike trails, and Clara will be looking to join a competitive football team.
What is your connection with the LISA mission, and where does it sit among your research activities?
I work on the detection and interpretation of gravitational waves, which are the ripples in spacetime generated by massive astrophysical objects, such as black holes, accelerating rapidly as they do in binary systems. These waves can also be described as travelling patterns of stretching and squeezing gravitational forces, not unlike the effect of lunar tides on the ocean.
LISA is a space-based gravitational-wave observatory jointly managed by the European Space Agency (ESA) and NASA, with ESA in the lead, and it's now planned for launch in 2035. It's the mission I've been waiting for since the beginning of my career! It was the subject of my first postdoc position, and it's the reason why I was at NASA/JPL as a research scientist: I served as the deputy project scientist for LISA, that is, the person at the interface between the engineers building the mission and the scientific community.
When NASA put aside LISA because of delays with the James Webb Space Telescope in 2011, other scientists and I redirected our attention towards other parts of the gravitational-wave spectrum. In my case, this meant going back to the ground-based detectors LIGO and Virgo, which I had worked on as a PhD student at the California Institute of Technology. When LIGO restarted operation after its upgrade, I became involved in the preparations for the data analysis and participated in the announcement of the first detection: I have great memories of that time.
In parallel, starting around 2012 I worked on the pulsar timing approach to gravitational-wave detection with the NANOGrav collaboration, which carries out observations at very low frequencies. NANOGrav is a nice project because it's interdisciplinary: you need a bit of everything, from neutron star physics to interstellar medium physics to data analysis – those were really good years too, where I worked intensely on statistical analysis tools as well as software. What makes me very happy is that my NANOGrav postdocs went on to land leadership positions in the field: it was great to be able to help train a new generation of scientists. In 2023 we finally had enough NANOGrav data to see a noise-like background of gravitational waves around 5nHz, probably coming from all the super-massive black-hole binaries in the local Universe. This result was a great satisfaction, especially as it came from a small team compared to LIGO: the first NANOGrav paper was written by six of us, and it really felt like my work.
After the 2015 LIGO detection, LISA was restarted in Europe and in the US, but it became increasingly clear to me that working on it from NASA would have more constraints than from other places like Switzerland. To build the Swiss data centre for LISA, we will be working with colleagues in countries including Germany, France and Great Britain. A sizable portion of hardware will be Swiss made too: ETH Space will take over the coordination of this work from the Department of Earth and Planetary Sciences. Once LISA flies in 2035, we'll be in the front row of LISA science and will gain incredible insight into dark objects across the Universe. I view LISA as the primary focus of my job here, but I'm also very invested in the opportunity to train young researchers and to teach again. Beyond LISA, I'm sure we'll come across other interesting topics to study: this is also why I called my group Gravitational Physics – it's partly a homage to Albert Einstein’s time at ETH, and it's a statement that we shouldn't limit ourselves to gravitational waves.
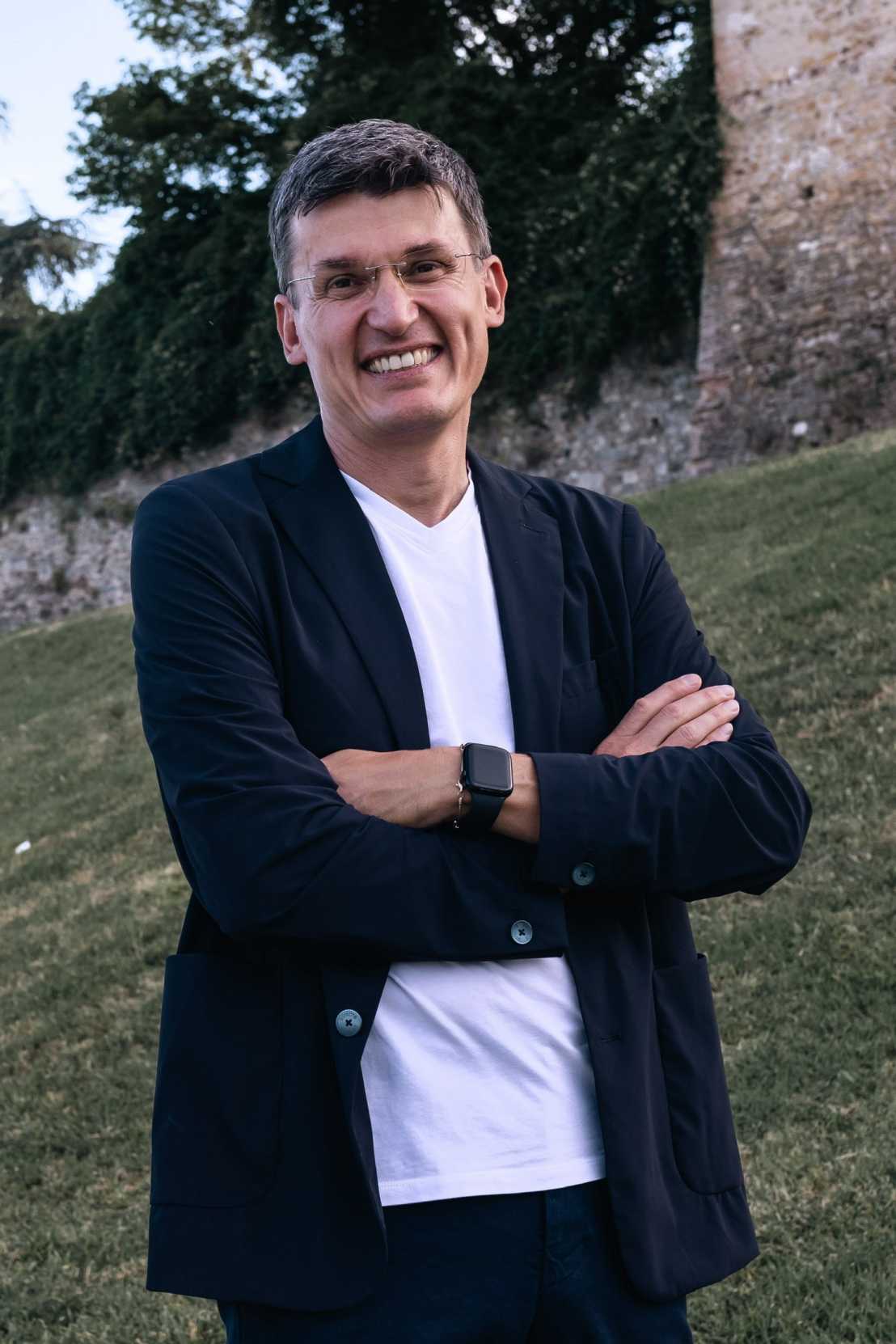
Based on what you said earlier, each experiment – LIGO, LISA and NANOGrav – covers a different range of frequencies in the detection of gravitational waves. What do you learn from each frequency interval?
The main point is that there's a correspondence between the detection frequencies and the observed sources of gravitational waves – the frequency corresponds in a pretty direct way to the source's mass. With a ground-based detector, you're around 100Hz and what you observe are stellar remnants, black holes and neutron stars, which is to say that you're in the range of sources with one to 100 solar masses. In fact, we didn't know that black holes could be as massive as 100 times our Sun! If you consider bigger black holes like the ones at the centre of our galaxy, you're around one million solar masses and you enter the LISA frequency range, which is in the mHz regime. You cannot detect these sources on Earth because seismic noise limits you to 10Hz or above – this is why moving to space and away from our planet is necessary. Being in space also means that you can build a bigger gravitational-wave detector, which makes the stretching and contracting of distances caused by gravitational waves more visible. If you go further down to the nHZ frequency range, you get access to super-massive black holes found inside larger galaxies. We won't observe their mergers because they take too long, but we can detect the continuous radiation from all of them by using pulsars, which are rotating, radio-emitting neutron stars, as time references. This is why NANOGrav detected a background, something like a vocal choir, as opposed to a single voice – in the future we may also be able to isolate single sources.
LIGO's first announcement of a gravitational-wave detection happened ten years ago. How did that event change the field?
To go from a promise for the future – we predict the existence of gravitational waves and work towards detecting them – to the status of experimental science in the Galilean sense – we experimentally observe sources and events that produce gravitational waves – was a crucial transition point. After 2015 I gave many talks where I said, finally I can show experimental data on my first slide! Ten years later, the catalogue of LIGO and Virgo counts almost 100 events – at this level you can do statistical analysis. The first event remains special, but there's value in all of them. We found out that black holes can be much heavier than we thought, and this was a real breakthrough. Then in 2017 there was an event that has so far remained unique: it was a neutron-star merger that was detected by LIGO and by astronomical observatories across the world ranging from optical, x-ray to gamma-ray wavelengths. We know that such neutron-star events are few compared to black-hole mergers because they're intrinsically fainter, so that was a lucky one.
Gravitational-wave astronomy is now a thriving research field, and we'll need many people to bring gravitational-wave detection away from Earth and into space. When you think about it, it's a story that starts in 1915 with Einstein's derivation of the gravitational wave equation. The first paper showing that gravitational waves have a physical effect, that they're not simply an artifact of reference frame and coordinate choice, came out in the fifties. At that time, researchers also detected the first black hole and developed lasers – two more steps along a century-long path that took us from mathematical calculations to a new kind of experimental astronomy. I think Einstein would have been so impressed!